Terpenes and alkaloids are more potent toxins and also simpler compounds than polyphenols and for these reasons they are more often took seriously in the analysis platforms that neglect especially tannins
Wide repertoire of analysis options for plant metabolites
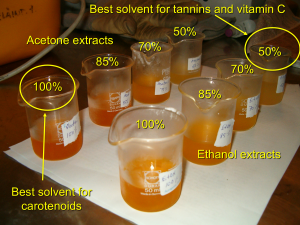
Figure 1. Different types of natural compounds require separate optimisation for their most efficient extraction protocol. Salminen et al. (2005) showed that when proanthocyanidin glycosides and vitamin C need to be effectively quantified from rose hip samples, their extraction should be done with 50% aqueous ethanol adjusted to pH 2 by 50 mM H3PO4. For carotenoids of rose hips that solvent was the worst of the solvents tested as can be even concluded from the extract colours above.
Although our primary focus at the Natural Chemistry Research Group is with the analysis of tannins and other polyphenols, we do quantify also other types of natural compounds from plants. So far these have included mainly terpenes and alkaloids, but also glucosinolates and cyanogenic glycosides have crossed our laboratory. We have collected some examples of these analysis schemes below.
Importance of the carotenoid analyses
Carotenoids are important plant pigments, since they assist chlorophyll in the harvesting of solar energy for the use of photosynthesis. For that reason they are produced in the chloroplast via the DXP pathway. Carotenoids cannot be made in herbivores or birds either, i.e. they need to receive their carotenoids from plants, either directly or indirectly. Some carotenoids are more essential than others, since e.g. various forms of vitamin A are derived from β-carotene. It has been shown that the depletion of carotenoid levels in the plasma can have many types of deletorious effects on health and even on mating success of birds.
Carotenoid analysis is a bit tricky, since they are easily degraded, if sample preparation protocols include lot of exposure to direct light or increased temperature. They are also highly lipophilic, meaning that their extraction needs to separately optimisation from the protocols meant for more polar metabolites (see Fig. 1). Furthermore, once injected into the LC column, care must be taken so that they are eluted out of the column. This is not necessarily a trivial task, since even pure acetonitrile may not be enough to elute all carotenes from the reversed-phase LC column, although all xanthophylls are quite easily eluted due to their more polar nature.
We at the Natural Chemistry Research Group have expertise in the analyses of carotenoids from plants (leaves and berries), insects (several species), birds (egg yolk, plasma, feathers) and sediments (lakes). Below we give out some examples of these analysis protocols, since so far we have analysed carotenoids with three different types of LC protocols, depending on the need and instrument available to us. Other examples are found in the section Chemical Ecology of Birds.
Carotenoid analyses with the optimized 4-solvent gradient
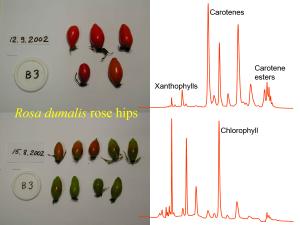
Figure 2. Rose hips have a relatively diverse carotenoid composition with xanthophylls such as lutein, carotenes such as β-carotene and lycopene, and carotene esters. Their analysis by HPLC-DAD at 450 nm with a Merck Purospher STAR RP-18 (55 × 2 mm, i.d., 3 µm) column can reveal also chlorophylls so that their ontogenic variation may be studied together with the carotenoids as shown above.
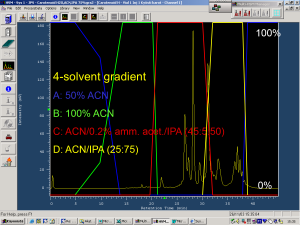
Figure 3. The 4-solvent LC-gradient used for the rose hip carotenoids. Carotenoid analyses with reversed-phase LC columns may require the use of stronger chromatographic solvents to acetonitrile (CAN). That alone may not be able to elute carotenes or especially carotene esters from the column. It can be seen that xanthophylls start to elute by 100% ACN at 15-22 minutes, but that increased percentages of isopropanol (IPA) are needed to elute the carotenes at 25-35 minutes and carotene esters at 35-40 minutes.
According to our knowledge, one of the most complex carotenoid compositions in plants can be found with rose hips. Moreover, the composition changes when the hips mature from green to red. Figure 2 shows how the early eluting xanthophylls in rose hips are accompanied by carotenes and even late-eluting carotene esters. These additional esters made it necessary to introduce a 4-solvent gradient for the LC analyses (Fig. 3) so that the early eluting xanthophylls could be nicely separated, then the carotenes and chlorophylls eluted before the esters are finally eluted with the strongest LC solvent of the gradient, i.e. isopropanol. This gradient is chromatographically excellent, but the use of isopropanol may cause leaking problems with the LC pump due to its low viscosity. Finally, the use of DAD detector with the carotenoid analyses is highly useful, since these compounds have really characteristic UV spectra that can be used to separate from all other types of natural compounds (Fig. 4).
Carotenoid analyses with the C30-column
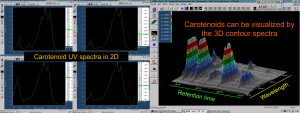
Figure 4. The UV spectra of carotenoids show a characteristic 3-maxima pattern at around 430-500 nm region. It can be used to differentiate them from all other natural compounds as visualised both the 2D-spectra (left) and 3D-contour spectra (right) recorded from the HPLC-DAD run of a rose hip carotenoid extract.
The slight problems related to the use of isopropanol can be avoided by changing the solvent to a bit more unusual LC eluent, acetone. This can be used together with a specific carotenoid column, e.g. the YMC C-30 (250 × 4 mm, i.d., 5 µm) column. With this column we have used both gradient and isocratic elution programs. Isocratic elution became possible by the 250 mm length of the column being able to retain the analytes well and by acetone being able to elute the analytes from the column. Otherwise isocratic elution programs would be impossible to utilize. Gradients need to be used with plant samples due to their more complex carotenoid composition, but since bird samples are simplified in their carotenoid profile, even isocratic elution is possible without compromising the separation of the analytes.
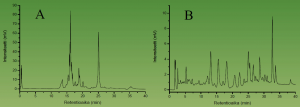
Figure 5. HPLC-DAD chromatograms of a carotenoid extract from the lake Köyliönjärvi sediment run with the YMC C-30 (250 × 4 mm, i.d., 5 µm) column. The non-optimised elution gradient on the left and the optimised one on the right. It can be seen that the optimised HPLC conditions allow much more acccurate analysis of tens of individual carotenoids, many of which are co-eluted with the non-optimised HPLC approach.
Figure 5 shows the carotenoid profiles of lake sediments achieved by the YMC C-30 column. We used this approach together with biologists and geologists to find out how the eutrophication processes have evolved during time in certain water bodies. This was possible by monitoring in various depths of the sediment the profile of such carotenoids that are specific to green algae. These types of algal populations typically grow in size when nutritive levels of the rivers and lakes increase. These studies have revealed e.g. that the eutrophication of the Lake Köyliönjärvi reached its peak during the 1970’s as evidenced by the accumulation of certain carotenoid pigments at the 15 cm depth of the sediment (Fig. 6).
Analysis of fingerprints for the volatile terpenes
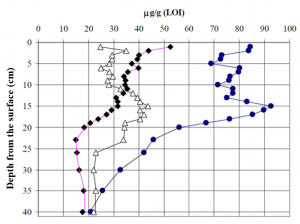
Figure 6. The profiles of the pigment concentrations in the Lake Köyliönjärvi sediment show that eutropication has been at its peak levels during the 1970’s that corresponds to the depth of 15 cm in the sediment.
Monoterpenes together with sesquiterpenes and diterpenes form the most important class of volatile natural compounds. They may function as signaling molecules between plants and even as cry-for-help molecules for plants that try to attract predators to take care of their foliar herbivores. The volatile cocktail of plants may thus be different depending on the induced state of the defense.
We at the Natural Chemistry Research Group have been interested in volatile terpenes as well to fingerprint the volatile cocktail of plant species. This is conveniently done by heating fresh plant tissue in water and collecting the volatiles from the headspace into of a drop of hexane hanging at the top of a glass of plastic capillary. Once the volatiles are absorbed into hexane, it can be taken back into the capillary and injected into GC-MS system for fingerprint analyses (Fig. 7).
Alkaloid analyses benefit from the use of high-resolution mass spectrometry
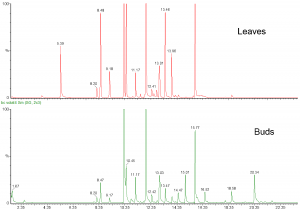
Figure 7. The GC-MS profiles of the volatile composition of the leaves and buds of Ribes nigrum. Many of the volatiles are common between both tissue types, but they also have their specific volatiles around 5 minutes, 15 minutes and 20 minutes.
Alkaloids are often considered as the most toxic group of plant natural compounds. Only some subgroups of triterpenes may match the toxicity levels obtained with the best of the alkaloids. For that reason it is understandable that this class of compounds is quite well covered in the studies of chemical ecology.
We at the Natural Chemistry Research Group have not tried to avoid alkaloid analyses, although we have tried to focus our analysis efforts to such compounds that would offer more challenges and would not be often quantified by others. For that reason alkaloids have not been our bread and butter. However, if we are interested of the chemical ecology of some specific plant species, genera or family, and those happen to produce also alkaloids, of course we take the whole chemical picture into account.
Such an example can be found from our efforts to characterize Ficus communities of Papua New Guinea as accurately as possible. In the paper by Volf et al. (2017) we quantified several individual alkaloids with Orbitrap high-resolution mass spectrometer. Figure 8 below shows how alkaloid contents and diversity showed variable patterns to polyphenols and triterpenoids in Ficus. Such results could not be achieved without looking at the individual alkaloids with the help of high-resolution mass spectrometry.
“Always start your phytochemical analysis by getting rid of tannins first.”
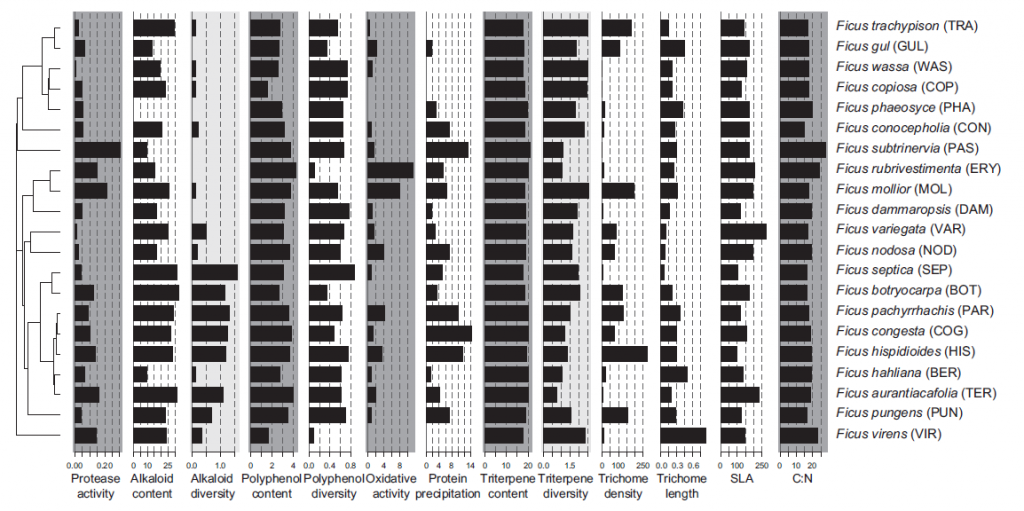
Figure 8. Distribution of the Ficus defences across the phylogeny. Note that alkaloid content and diversity differ from those obtained for polyphenols and triterpenes. The same is true for functional properties of Ficus, such as oxidative activity and protein precipitation capacity. More details can be found at Volf et al. (2017).